Chemokine Oligomerization & GAG Interactions
Overall, our laboratory is interested in studying the complex and dynamic interactions of chemokines with themselves (through oligomerization), with GAGs, and with receptors that together coordinate the multistep nature of cell migration in vivo. We take an interdisciplinary approach using a combination of structure (NMR, crystallography), biophysics (Surface Plasmon Resonance, other), biochemistry/protein engineering, and cell biology to understand the molecular basis of cell migration.
Oligomerization. Much is know about the structures of chemokines from NMR and crystallography studies by our lab and many others. Paradoxically, despite their diversity of functions, most chemokines have similar tertiary folds (see Figure 1). However many chemokines form dimers in solution, the nature of which differs between families (CC, CXC, CX3C, XC). For the most part, the CXC family chemokines dimerize with structures resembling IL-8/CXCL8. By contrast, most CC family chemokine dimers form elongated structures like MCP-1/CCL2 (Figure 1). In addition to dimers, several chemokines form higher order oligomers. Although the monomeric form is the form that binds to the receptor to induce cell migration [1], oligomerization is required for function in vivo [2]. In fact, engineered monomers are not only non-functional in vivo, but they can block the function of the WT protein and therefore have potential therapeutic value [3]. Chemokine oligomerization may have important roles in some signaling processes, and chemokines also form hetero-oligomers [4]. Thus structures of chemokine homo- and hetero-oligomers and their functional role are major interests of our laboratory.
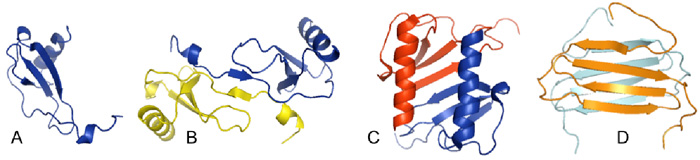
Figure 1: A. The monomer structure of MCP-1/CCL2 illustrates a typical chemokine fold. B. Structure of the MCP-1/CCL2 dimer [5] . C. Structure of the IL-8/CXCL8 dimer [6]. D. Structure of the non-canonical dimer of the chemokine lymphotactin/XCL1 [7,8].
Chemokine:GAG Interactions. In addition to possible roles in signaling, oligomerization is involved in a second interaction -- binding to GAGs [2]. GAGs are structurally diverse linear carbohydrates that are often attached to membrane bound protein anchors as proteoglycans. Protein:GAG interactions have important roles in protein structure, assembly, signaling, regulation, and they impact biology from development to disease. Cells even change their carbohydrate coats when they become cancerous or inflamed. In the context of the chemokine network, chemokine:GAG interactions are involved in locally sequestering chemokines on cell surfaces, preventing diffusion so that chemokines provide directional cues for migrating cells. Other potential roles for chemokine:GAG interactions include transport across cells, regulation of oligomerization with as yet poorly characterized signaling consequences, and regulation of receptor binding and activation. Since GAG structures are highly diverse, interactions with chemokines may contribute to the specificity and fine tuning of cell migration, well beyond the chemokine:receptor interaction.
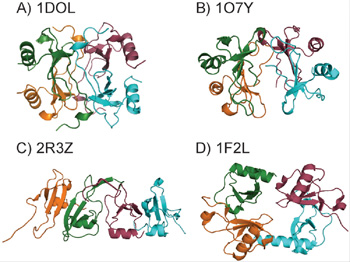
Figure 2. A. Structure of the MCP-1 Tetramer [10]. B. Structure of one of the three tetramer structures of human IP-10 [11]. C. Structure of the murine IP-10 tetramer [12]. D. Structure of the fractalkine tetramer [13].
At present, there is little structural information about chemokine:GAG interactions and their precise functional consequences, a major interest of the laboratory. However, it is known that binding to GAGs causes many chemokines to oligomerize beyond dimers; for example, MCP-1 forms tetramers in the presence of a heparin octasaccharide [9]. Tetramers of other chemokines have also been captured by crystallography, and may represent forms stabilized upon GAG-binding (Figure 2). As with chemokine oligomerization, we are interested in the structures of chemokine:GAG complexes and their functional roles. One of our hypotheses is that structural plasticity through oligomerization of chemokines on GAGs, or GAG-induced folding of unstructured domains, are mechanisms of achieving functional specificity/diversity, and that through changes in oligomerization or unfolded state structure, a given chemokine may recognize different GAGs.
Paavola C, Hemmerich S, Grunberger D, Polsky I, Bloom A, Freedman R, Mulkins M, Bhakta S, McCarley D, Wiesent L, Wong B et al: Monomeric monocyte chemoattractant protein-1 (MCP-1) binds and activates the MCP-1 receptor CCR2B. J Biol Chem (1998) 273:33157-33165.
Proudfoot AEI, Handel TM, Johnson Z, Lau EK, LiWang P, Clark-Lewis I, Borlat F, Wells TNC, Kosco-Vilbois MH: Glycosaminoglycan binding and oligomerization are essential for the in vivo activity of certain chemokines. Proceedings of the National Academy of Sciences of the United States of America (2003) 100:1885-1890.
Handel TM, Johnson Z, Rodrigues DH, Dos Santos AC, Cirillo R, Muzio V, Riva S, Mack M, Deruaz M, Borlat F, Vitte PA et al: An engineered monomer of CCL2 has anti-inflammatory properties emphasizing the importance of oligomerization for chemokine activity in vivo. J Leukoc Biol (2008) 84:1101-1108.
Crown SE, Yu Y, Sweeney MD, Leary JA, Handel TM: Heterodimerization of CCR2 chemokines and regulation by glycosaminoglycan binding. J Biol Chem (2006) 281:25438-25446.
Handel TM, Domaille PJ: Heteronuclear (1H, 13C, 15N) NMR assignments and solution structure of the monocyte chemoattractant protein-1 (MCP-1) dimer. Biochemistry (1996) 35:6569-6584.
Clore GM, Appella E, Yamada M, Matsushima K, Gronenborn AM: Three-dimensional structure of interleukin 8 in solution. Biochemistry (1990) 29:1689-1696.
Kuloglu ES, McCaslin DR, Markley JL, Volkman BF: Structural rearrangement of human lymphotactin, a C chemokine, under physiological solution conditions. J Biol Chem (2002) 277:17863-17870.
Tuinstra RL, Peterson FC, Kutlesa S, Elgin ES, Kron MA, Volkman BF: Interconversion between two unrelated protein folds in the lymphotactin native state. Proc Natl Acad Sci U S A (2008) 105:5057-5062.
Lau EK, Kungl A, Paavola C, Johnson Z, Borlat F, Proudfoot AE, Handel TM: Identification of the Glycosaminoglycan Binding Site of the CC Chemokine, MCP-1; Implications for Structure and Function In Vivo. J Biol Chem (2004) 279:22294-22305.
Lubkowski J, Bujacz G, Boque L, Domaille PJ, Handel TM, Wlodawer A: The structure of MCP-1 in two crystal forms provides a rare example of variable quaternary interactions. Nat Struct Biol (1997) 4:64-69.
Swaminathan GJ, Holloway DE, Colvin RA, Campanella GK, Papageorgiou AC, Luster AD, Acharya KR: Crystal structures of oligomeric forms of the IP-10/CXCL10 chemokine. Structure (Camb) (2003) 11:521-532.
Jabeen T, Leonard P, Jamaluddin H, Acharya KR: Structure of mouse IP-10, a chemokine. Acta Crystallogr D Biol Crystallogr (2008) 64(Pt 6):611-619.
Hoover DM, Mizoue LS, Handel TM, Lubkowski J: The crystal structure of the chemokine domain of fractalkine shows a novel quaternary arrangement. J Biol Chem (2000) 275:23187-23193.